[ad_1]
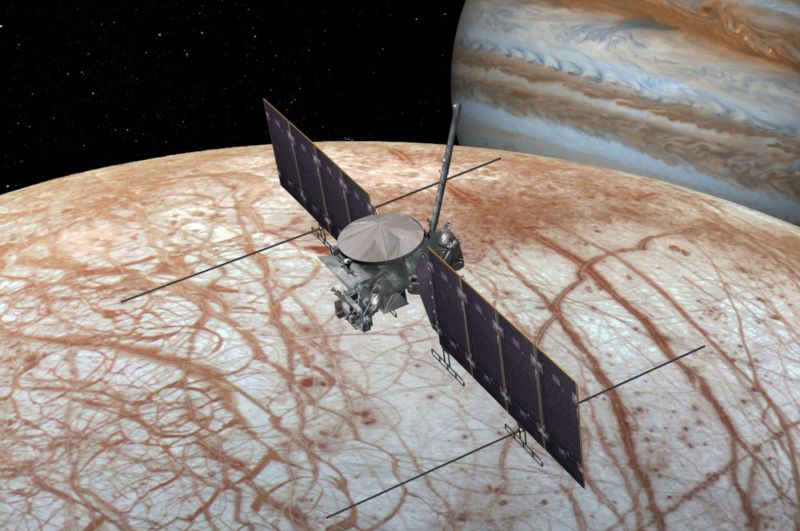
If all goes to plan, 2024 will see NASA launch the Europa Clipper program, designed to take multiple steps to study the water-rich moon’s potential to host life. The big challenge the mission will face is that liquid water is likely to be far below Europa’s frozen surface. At best, we can hope for some indication of what’s going on based on the composition of any material trapped in the ice itself or the possible presence of geysers releasing pieces of its interior into space.
This makes it essential to understand the kind of remote sensing that might be possible. And to that end, NASA scientists have looked at how ice behaves in Jupiter’s high-radiation environment. Scientists have found that Europa’s ice likely glows in the dark, and that glow could hold some information about what’s in the ice.
Let there be light
The mechanism that can make Europa’s ice glow is a bit like the one used by a black light poster. There, light outside the visible wavelengths excites the molecules which then release the energy at the wavelengths we can see. In the case of Europa, the excitation energy does not come from light, but that energy is indirectly powered by Jupiter’s magnetic fields. These fields collect charged particles released by the planet itself (or one of its moons) and accelerate them. (Much of the material in Jupiter’s high-radiation areas was ejected into space by volcanoes on its moon Io.)
Some of these charged particles will strike Jupiter’s moons and deposit energy into the material on their surface. Some of that energy will undoubtedly come out as photons; the question is what energy photons have, which will determine how readily we can detect them.
So, NASA’s Murthy Gudipati and Bryana Henderson reunited with Fred Bateman at the National Institute of Standards and Technology, and did what anyone with access to the right toys would do: They froze the ice up to 100 Kelvin and bombed it. of electrons. And then they froze various combinations of salts and tried to see if that changed anything.
Many wavelengths
Using the water sample alone, Gudipati et al. determined that the ice will glow in the dark at wavelengths visible under these conditions. While the peak lies on the border between the blue and green wavelengths, it is very broad with a broad shoulder at the redder end of the spectrum. This means that the glow of the samples looks quite white. The light goes out as soon as the radiation is interrupted and increases in intensity if the electronic radiation is brought to higher energies.
The comparison with the salts showed mainly that the salts interfered with this glow. But the salts did not interfere with all parts evenly. For example, sodium sulfate causes the bluer end of the spectrum to drop by raising the red end slightly. Sodium chloride, on the other hand, uniformly decreases the entire spectrum. Sodium carbonate eliminates virtually all emissions except those near infrared. The big exception was magnesium sulfate, which seemed to increase most of the spectrum, especially in the green to red region.
Read the light
These differences in salt content suggest that it might be possible to deduce things about the composition of Europa’s ice (and thus the ocean below) by reading its glow.
As a first step, the researchers examine some of the images made by ground-based telescopes that include the unlit side of Europa. Unfortunately, these images are dominated by the glow of the radiation hitting the faint gases that are weakly retained by Europa’s gravity. This is a problem for imaging from Earth, but it turns out that the radiation won’t be a problem for the Europa Clipper, which will make some orbital shifts that will take it between the source of this radiation and the moon’s surface.
Then, the three researchers obtained all available information about the instruments the Europa Clipper will carry and tried to estimate whether the Clipper could pick up the glowing ice on the surface.
They decided the wide-angle camera was the best solution. While it has a relatively low per-pixel resolution (meaning the camera will struggle to associate specific compositions with landscape features), the trade-off is that the Clipper will be able to gather more light in each pixel. The researchers calculate how much light an area over Europa should generate given the typical radiation power in the environment and how many of those photons would reach the camera. This turns out to be in the area of 100,000 photons per second for pure water ice, which is about 100 times higher than the photons produced by the radiation hitting the material in Europa’s orbit.
This is when we introduce a whole host of caveats. For one, some of the filters on the camera cut mean that some wavelengths that might otherwise help determine the composition will end up being grouped in the same data container. The other thing is that the ice in these test cases is made up of unusually pure water, with only one salt in it. Real-world Europe will likely have more than one salt present and is likely to have a complicated mix of organic materials collectively called tholins here I’m. So, it is probably worth checking whether these affect emissions in any way.
Sulfur, oxygen and hydrogen
The last thing, mentioned by the authors themselves, is that electrons are certainly not the only things that bombard ice. As mentioned earlier, much of the material displaced by Jupiter’s magnetic field includes ions of sulfur, oxygen, and hydrogen. These may impact the ice in different ways, which people may also want to explore.
However, the mission isn’t even expected to launch for a few years, so there’s plenty of time to start fixing this problem. And since it might be hard to imagine anything else when the camera is only 50km above the dark side of Europe, there is no obvious harm in collecting the data we want to analyze, even if we don’t have exactly what it might fully say. solved yet.
Nature Astronomy, 2020. DOI: 10.1038 / s41550-020-01248-1 (About DOI).
Source link