[ad_1]
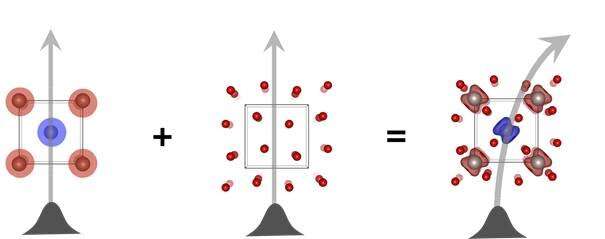
The electrons (gray wave packets) in the antiferromagnetic (left) and non-magnetic (center) crystals move along the applied electric current (right). The combination of antiferromagnetic and non-magnetic atoms (right) generates a surprisingly transverse Hall motion of the electron. In the left and right panels, blue and red shades mark the positive and negative magnetization densities. Credit: Libor Šmejkal
Sometimes combinations of different things produce effects that no one expects, for example when completely new properties appear that the two combined parts do not have on their own. Dr. Libor Šmejkal of Johannes Gutenberg University of Mainz (JGU) found such an unexpected property: he combined antiferromagnetic substances with non-magnetic atoms and found that contrary to current doctrine, a Hall current occurs, which is not the case of both antiferromagnetics or non-magnetic substances individually.
This could offer entirely new potential for nanoelectronics. On the one hand, these material combinations occur very frequently in nature. Therefore, this discovery has the potential to reverse the growing demand for rare heavy elements in conventional magnetoelectronics and, instead, direct research and applications towards abundant materials. Furthermore, the Hall current exhibits low energy dissipation. This is particularly important in light of the fact that information technology is becoming the largest consumer of energy in industries. Since the materials do not have an outward magnetic field and are therefore magnetically invisible, they can be packed very tightly and allow for a high degree of miniaturization of nanoelectronics. These previously overlooked materials also score in terms of speed since they allow for many times faster speeds than ferromagnets, so frequencies could be shifted from the gigahertz range to the terahertz range. In short: The discovery holds a special place in the rapidly growing new field of antiferromagnetic magnetoelectronics, also known as spintronics. Dr. Libor Šmejkal and his colleagues from the University of Mainz recently published their results in Advances in science.
What is the Hall current?
To understand Šmejkal’s research, one must start with the Hall effect which is named after physicist Professor Edwin Hall. If a voltage is applied to conventional non-magnetic conductors such as copper, the current flows in the direction given by the electric field. However, if an external magnetic field is added, the current bends away from the applied direction. This additional cross component is known as the Hall current. The Hall effect described was used to characterize semiconductors, which have shaped modern silicon electronics. Hall’s second discovery: the internal magnetization of a ferromagnetic conductor such as iron can also lead to such a deflection of the cross current. This has also made the Hall effect one of the cornerstones of magnetoelectronics, a broad field extending from sensor to memory technologies.
The discovery of antiferromagnets, which are much more common in nature than ferromagnets, is attributed to Professor Louis Néel. In these the magnetic moments of the atoms are oriented in opposite directions. The effects observed in ferromagnets then cancel each other out, including the Hall current. The antiferromagnets behave towards the outside like the usual non-magnetic conductors and are therefore not applicable for magnetoelectronics.
Unusual effect: Hall current in antiferromagetes
Non-magnetic and antiferromagnetic crystals have been known for decades for the absence of Hall currents. Dr Libor Šmejkal, however, found a crystal with an intriguing combination of non-magnetic and antiferromagnetic atoms that produces a strong Hall current. Surprisingly, crystals with antiferromagnetic and non-magnetic atoms are not rare in nature, but rather widespread.
‘Breaking with conventional scientific wisdom requires extraordinary talents and skills,’ said the research team’s director, Professor Jairo Sinova. “This is also the case with Dr. Libor Šmejkal. He is an exceptional physical talent who, as a recent doctorate, already enjoys a reputation as an international leader in his field.”
Šmejkal defended his PhD. thesis only a few months ago, but has already given a dozen invited lectures at international conferences and published various articles in high quality scientific journals. Shortly after his defense PhD, Šmejkal assumed the position of an independent team leader in the INSPIRE group at the JGU Institute of Physics.
Antiferromagnets demonstrate their potential for spin-based information technology
Libor Šmejkal et al. Break of the crystal time inversion symmetry and spontaneous Hall effect in collinear antiferromagnets, Advances in science (2020). DOI: 10.1126 / sciadv.aaz8809
Provided by Universitaet Mainz
Quote: Antiferromagnets are suitable for nanoelectronics without dissipation, contrary to current theories (2020, November 9) retrieved on November 9, 2020 from https://phys.org/news/2020-11-antiferromagnets-suitable-dissipationless-nanoelectronics-contrary .html
This document is subject to copyright. Aside from any conduct that is correct for private study or research purposes, no part may be reproduced without written permission. The content is provided for informational purposes only.
[ad_2]
Source link