[ad_1]
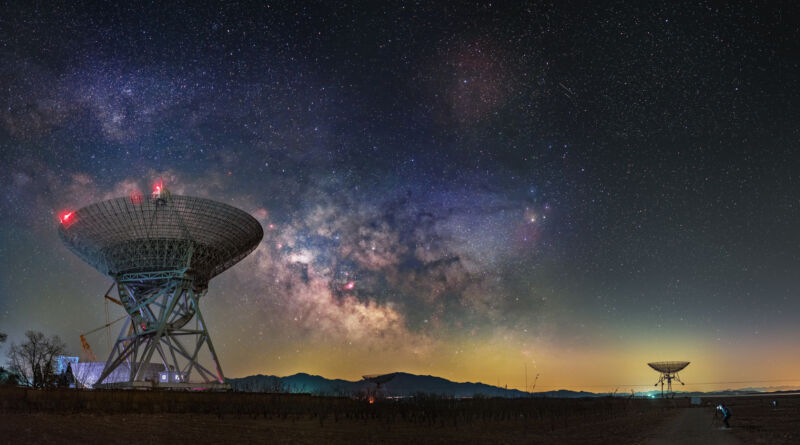
Haitong Yu | Getty Images
If Jason Benkoski is right, the path to interstellar space begins in a shipping container hidden behind a high bay at the lab in Maryland. The set-up looks like something out of a low-cost science fiction film: one wall of the container is lined with thousands of LEDs, an inscrutable metal trellis runs in the center and a thick black curtain partially obscures the apparatus. This is the solar simulator from the Johns Hopkins University Applied Physics Laboratory, an instrument that can glow with the intensity of 20 suns. On Thursday afternoon, Benkoski mounted a small black and white tile on the truss and pulled a dark curtain around the setup before exiting the container. Then he hit the light switch.
Once the solar simulator got hot, Benkoski started pumping liquid helium through a small built-in tube that snaked through the plate. The helium absorbed heat from the LEDs as it wound through the channel and expanded until it was finally released through a small nozzle. It might not sound like much, but Benkoski and his team have just demonstrated solar thermal propulsion, a previously theoretical type of rocket engine powered by the sun’s heat. They think it could be the key to interstellar exploration.
“It’s really easy for someone to dismiss the idea and say, ‘On the back of an envelope, it looks great, but if you really build it, you’ll never get those theoretical numbers,'” says Benkoski, a Materials scientist at the Applied Physics Laboratory. and the team leader working on a solar thermal propulsion system. “What this is showing is that solar thermal propulsion is not just a fantasy. It might actually work. “
Only two spacecraft, Voyager 1 and Voyager 2, have left our solar system. But this was a scientific bonus after completing their main mission to explore Jupiter and Saturn. Neither spacecraft was equipped with the right tools to study the boundary between our star’s planetary fiefdom and the rest of the universe. Plus, the Voyager twins are slow. Trudging along at 30,000 miles per hour, it took them nearly half a century to escape the influence of the sun.
But the data they sent back from the edge is tempting. It showed that much of what physicists had predicted about the environment at the edge of the solar system was wrong. Unsurprisingly, a large group of astrophysicists, cosmologists and planetary scientists are clamoring for a dedicated interstellar probe to explore this new frontier.
In 2019, NASA contacted the Applied Physics Laboratory to study concepts for a dedicated interstellar mission. Late next year, the team will present its research to the Heliophysics ten-year survey of the National Academies of Sciences, Engineering and Medicine, which determines the scientific priorities related to the sun for the next 10 years. APL researchers working on the Interstellar Probe program are studying all aspects of the mission, from cost estimates to instrumentation. But simply figuring out how to get to interstellar space in a reasonable amount of time is by far the biggest and most important piece of the puzzle.
The boundary of the solar system, called the heliopause, is extremely far away. When a spacecraft reaches Pluto, it is only one third of the way to interstellar space. And the APL team is studying a probe that would travel three times beyond the edge of the solar system, a 50 billion-mile journey, in about half the time it takes the Voyager probe alone to reach the edge. To carry out that kind of mission, they will need a probe unlike anything ever built. “We want to create a spacecraft that goes faster, farther and gets closer to the sun than it ever has before,” says Benkoski. “It’s like the hardest thing you could do.”
In mid-November, Interstellar Probe researchers met online for a week-long conference to share updates as the study enters its final year. At the conference, teams from APL and NASA shared the results of their work on thermal solar propulsion, which they believe is the fastest way to get a probe into interstellar space. The idea is to power a rocket engine with heat from the sun rather than combustion. According to Benkoski’s calculations, this engine would be about three times more efficient than the best conventional chemical engines available today. “From a physics standpoint, it’s hard for me to imagine anything that can beat solar thermal propulsion in terms of efficiency,” says Benkoski. “But can you stop it from exploding?”
Unlike a conventional engine mounted on the aft end of a rocket, the solar thermal engine the researchers are studying would be integrated with the spacecraft’s shield. The rigid flat shell consists of a black carbon foam with one side coated in a white reflective material. Externally it would be very similar to the Parker Solar Probe heat shield. The key difference is the winding piping hidden just below the surface. If the interstellar probe passes close to the sun and pushes the hydrogen into the vascular system of its shield, the hydrogen will expand and explode from a nozzle at the end of the tube. The heat shield will generate thrust.
It’s simple in theory, but incredibly difficult in practice. A solar thermal rocket is only effective if it can perform an Oberth maneuver, an orbital mechanical attack that turns the sun into a giant slingshot. The sun’s gravity acts as a force multiplier that greatly increases the speed of the aircraft if a spacecraft fires its engines while circling the star. The closer a spacecraft gets to the sun during an Oberth maneuver, the faster it will go. In the design of the APL mission, the interstellar probe would pass only a million miles from its murky surface.
To put this in perspective, by the time NASA’s Parker solar probe makes its closest approach in 2025, it will be within 4 million miles of the sun’s surface and will book it at nearly 430,000 miles per hour. This is about twice the speed the interstellar probe aims to hit, and the Parker Solar Probe has gained speed with the gravitational assistance of the Sun and Venus over the course of seven years. The interstellar probe will have to accelerate from about 30,000 miles per hour to about 200,000 miles per hour in one swipe around the sun, which means getting closer to the star. Really close.
Approaching a thermonuclear explosion the size of a sun creates all sorts of material challenges, says Dean Cheikh, a materials technologist at NASA’s Jet Propulsion Laboratory who presented a case study on the solar thermal rocket at the recent conference. For the APL mission, the spacecraft spent approximately two and a half hours in temperatures of approximately 4,500 degrees Fahrenheit while completing the Oberth maneuver. It’s more than hot enough to melt through the Parker Solar Probe’s heat shield, so Cheikh’s team at NASA found new materials that could be coated on the outside to reflect heat energy. Combined with the cooling effect of hydrogen flowing through channels in the heat shield, these coatings would keep the interstellar probe cool while being struck by the sun. “You want to maximize the amount of energy you are returning,” Cheikh says. “Even small differences in the reflectivity of the material start to significantly heat up your spacecraft.”
An even bigger problem is how to manage the hot hydrogen flowing through the channels. At extremely high temperatures, the hydrogen would eat through the carbon-based core of the heat shield, which means the inside of the channels will need to be coated with a stronger material. The team identified some materials that could do the job, but there isn’t much data on their performance, especially the extreme temperatures. “There aren’t many materials that can meet these needs,” says Cheikh. “In a way that’s okay, because we just have to look at these materials. But it’s also bad because we don’t have many options. “
The big result of his research, Cheikh says, is that there are many tests to be done on the materials of the heat shield before a solar thermal rocket is sent around the sun. But it’s not a puzzle. Indeed, the incredible advances in materials science make the idea finally feasible more than 60 years after it was first conceived by U.S. Air Force engineers. “I thought I had this great idea independently, but people were talking about it in 1956,” says Benkoski. “Additive manufacturing is a key component of this and we couldn’t have done it 20 years ago. Now I can 3D print metal in the lab. “
While Benkoski wasn’t the first to pitch the idea of a solar thermal propulsion, he believes he is the first to demonstrate a prototype engine. During his experiments with the tile channeled into the container, Benkoski and his team showed that it was possible to generate thrust by using sunlight to heat a gas as it passed through conduits embedded in a heat shield. These experiments had several limitations. They did not use the same materials or propellants that would have been used on an actual mission, and the tests occurred at temperatures well below what an interstellar probe would experience. But the important thing, says Benkoski, is that the data from the low-temperature experiments matched models that predict how an interstellar probe would behave in its actual mission once adjustments are made for the different materials. “We did it on a system that would never be able to fly. And now the second step is that we start replacing each of these components with the material that you would put on a real spacecraft for an Oberth maneuver, ”says Benkoski.
The concept still has a long way to go before it’s ready to be used on a mission, and with only a year left in the Interstellar Probe studio, there isn’t enough time to launch a small satellite to experiment in low earth orbit. But by the time Benkoski and his colleagues from the APL present their report next year, they will have generated a wealth of data that will lay the foundation for testing in space. There is no guarantee that the National Academies will select the interstellar probe concept as a top priority for the next decade. But whenever we’re ready to leave the sun behind, there’s a good chance we’ll have to use it for a boost on our way out.
This story originally appeared on wired.com.
Source link