[ad_1]
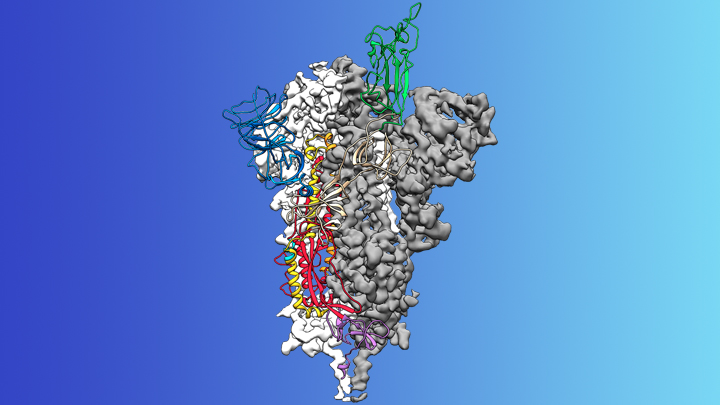
When the COVID-19 pandemic was first recognized for the threat it is, researchers were quick to find something that could block the spread of the virus. While vaccines have attracted much attention lately, there was also hope that a therapy could be developed that could block the worst effects of the virus. Most of these have been extremely practical: identifying enzymes essential for virus replication and testing drugs that block similar enzymes from other viruses. These drugs are designed to be relatively easy to store and administer and, in some cases, have already been tested for safety in humans, making them reasonable choices for quickly getting something ready for use.
But the tools we have developed in biotechnology allow us to do far less practical things, and a paper released today describes how they can be used to inactivate SARS-CoV-2. This is by no means a path to practical therapy, but it does provide a fantastic window into what we can achieve by manipulating biology.
Throw it in the trash
The entire effort described in the new article focuses on a simple idea: if you understand how to destroy one of the virus’s key proteins, it won’t be able to infect anything. And, conveniently, our cells have a way to destroy proteins, as that’s often a useful thing to do. In some cases, the proteins that are destroyed are damaged; in others, proteins are produced and destroyed at high rates to allow the cell to respond rapidly to changing conditions. In some cases, changes in the environment or activation of signaling pathways can trigger widespread destruction of proteins, allowing the cell to rapidly alter its behavior.
This system is based on a small protein called “ubiquitin”. When a protein needs to be targeted for destruction, enzymes called ubiquitin ligases chemically bind a chain of ubiquitins to it. These serve as a tag recognized by the enzymes that digest the proteins to which ubiquitin is attached.
So, the idea behind the new work is to identify a key viral protein and figure out how to attach ubiquitin to it. The cell would then take care of the rest, digesting the viral protein and thus blocking the production of any useful viruses in that cell. In this case, the researchers decided to target the spike protein that sits on the surface of coronaviruses and allows them to attach and infect new cells.
Unfortunately, there are no proteins that bind ubiquitin to the viral peak protein. Or, rather, there were no proteins that matched that description.
But a Harvard team has now produced one.
Bioengineering
The team’s method of doing this began with the fact that we know something that attaches to the viral peak protein: the cellular protein that it attaches to to enter the cell. This is called angiotensin 2 converting enzyme, or ACE2, but we will call it green protein because it is the color we use in this diagram. The idea was to find a portion of this protein that attached to the peak (aka the red protein) and link it to a protein that adds ubiquitin (blue). It sounds simple enough.
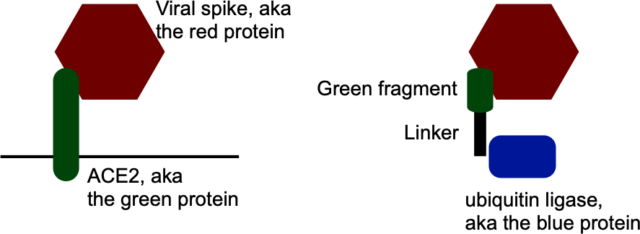
John Timmer
But there is a complication: the green protein also attaches itself to other proteins found on healthy, uninfected cells. So, if you’re not careful, your virus-destroying enzyme will also end up destroying proteins that are essential for the health of uninfected cells. That would be a pretty big “oopsie”.
To solve this problem, the researchers downloaded data that showed atomic-level details of the structure of red and green proteins, as well as how these proteins interact. (Yes, it is available.) Then they transferred this data into a software package that finds the most energetically favorable interactions between proteins. (Yes, those do exist.) They asked the program to virtually cut out the green protein and find smaller pieces that met two conditions: the pieces attached to the virus’s red protein but not to the one found on the surface of healthy human cells.
Once they identified a specific part of the red in the green protein, the researchers fused it with something that attached itself to the blue protein, which would link ubiquitin to red. This hybrid would act as a bridge, linking the viral red protein to a blue one that would bind ubiquitin.
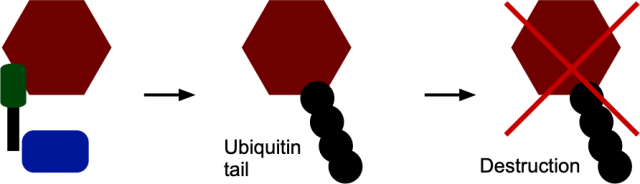
John Timmer
It worked, but not particularly well. The authors linked the peak (the red one) to a fluorescent protein and found that making their hybrid protein reduced fluorescence by about 30 percent. Better than nothing, but not great.
Optimization
So how to make it better? The researchers used the software package to create mutations at each individual location in their green protein fragment and checked what each did for its affinity for the viral spike protein. Anything that looked promising was engineered into real protein. One of these greatly improved performance; now, instead of lowering the fluorescence by 30%, it has dropped by 50%.
But that was not the end of their efforts. The green fragment / linker hybrid they built served as a bridge by attaching to both the red and blue protein that binds ubiquitin. To further increase efficiency, the researchers simplified things a bit by directly connecting the blue enzyme to the green fragment. With this in place, there is a direct link between the protein that the red attaches to and the blue one that guarantees its destruction. This reduced the amount of fluorescent spike proteins present in the cells by 60%.
So, a great application of biotechnology, right? Unfortunately, it’s also likely to be absolutely useless, and not just because we don’t know if a 60% reduction is significant. For this to be effective, it should be produced by the cells as they have active infections. Which means we have to insert the gene that encodes the protein they built into the cells, at least temporarily. We can definitely do it – it’s the technology that some of the leading vaccine candidates rely on. But to make a vaccine work, we don’t need to get one gene that’s active in so many cells. To protect an entire organ, we could.
The bottom line: This is likely not a start, especially considering there are promising vaccines and many other potential therapies awaiting safety testing. However, the things that make this type of technology extremely impractical for treating a virus in humans may not apply to other use cases like bacteria, crops, animals, or even less urgent medical needs. So while the details of this work aren’t really meaningful, it’s worth keeping in mind that we’ve developed all the underlying technology needed.
Communications Biology, 2020. DOI: 10.1038 / s42003-020-01470-7 (About DOI).
Source link