[ad_1]
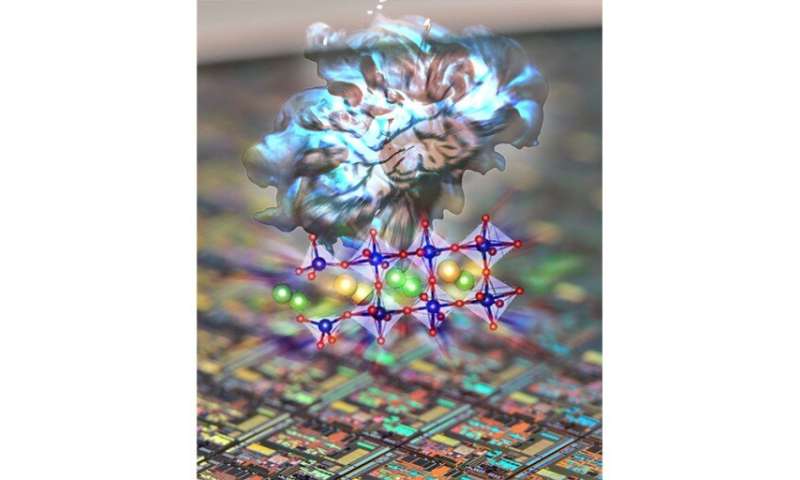
Ball and stick (central) representation of the defective material simulated in the study, for neuromorphic applications. Credit: Illustration by Emmanuel Gygi
Even with decades of unprecedented development in computing power, the human brain still retains many advantages over modern computer technologies. Our brains are extremely efficient for many cognitive tasks and do not separate memory and computers, unlike standard computer chips.
In the last decade, the new paradigm of neuromorphic computing has emerged, inspired by the neural networks of the brain and based on energy-efficient hardware for processing information.
To create devices that mimic what happens in the neurons and synapses of our brains, researchers must overcome a fundamental molecular engineering challenge: how to design devices that exhibit a controllable and energy-efficient transition between different resistive states triggered by stimuli. arriving.
In a recent study, scientists from the University of Chicago Pritzker School of Molecular Engineering (PME) were able to predict the design rules for such devices.
Published on 10 November in npj Computational Materials, the study predicted novel ways of engineering and triggering changes in electronic properties in different classes of transition metal oxides, which could be used to form the basis of neuromorphic computing architectures.
“We used quantum mechanical calculations to unravel the mechanism of the transition, highlighting exactly how it occurs on the atomistic scale,” said Giulia Galli, Liew Family Professor at Pritzker Molecular Engineering, professor of chemistry and co-author of the study. “We also devised a model to predict how to trigger the transition, showing good agreement with available measurements.”
The impact of defects on electronic properties
The researchers studied oxide materials that show a change in electronic properties from a metal – which conducts electricity – to an insulator – which does not allow electricity to pass – with varying concentrations of defects. Defects can be missing atoms or some impurities that replace the atoms present in a perfect crystal.
To understand how defects change the state of the material from metal to insulator, the authors calculated the electronic structure at different concentrations of defects using methods based on quantum mechanics.
“Understanding the intricate charge interdependence of these defects, how atoms rearrange themselves in the material, and how spin properties vary is crucial to controlling and ultimately triggering the desired transition,” said Shenli Zhang. UChicago postdoctoral researcher and first author of the paper.
“Compared to traditional semiconductors, the oxide materials we studied require much less energy to switch between two completely different states: from a metal to an insulator,” continued Zhang. “This feature makes these materials promising candidates for use as artificial neurons or artificial synapses for large-scale neuromorphic architectures.”
The study, published by Zhang and Galli, was conducted within the Quantum Materials for Energy Efficient Neuromorphic Computing (QMEENC) research center, funded by the Department of Energy and led by Prof. Ivan Schuller of UC San Diego.
“Understanding quantum materials will provide key solutions to many scientific and technological problems, including reducing energy consumption in computational devices,” said Schuller. “Given the complexity of quantum materials, the Edisonian approach of trial and error is no longer feasible and quantitative theories are needed.”
Such high-level theories are computationally demanding and have been the goal of a long line of work.
“First principles calculations are playing a key role in driving the molecular engineering of neuromorphic computation. It is exciting to see the methods we have been developing for years come to fruition,” said Galli.
Solving material problems with a quantum computer
Shenli Zhang et al, Understanding the metal-to-insulator transition in La1 – xSrxCoO3 – δ and its applications for neuromorphic computation, npj Computational Materials (2020). DOI: 10.1038 / s41524-020-00437-w
Provided by the University of Chicago
Quote: Scientists Uncover Secrets to Designing Brain-Like Devices (2020, November 10) Retrieved November 10, 2020 from https://phys.org/news/2020-11-scientists-uncover-secrets-brain-like- devices.html
This document is subject to copyright. Apart from any conduct that is correct for private study or research purposes, no part may be reproduced without written permission. The content is provided for informational purposes only.
[ad_2]
Source link