[ad_1]
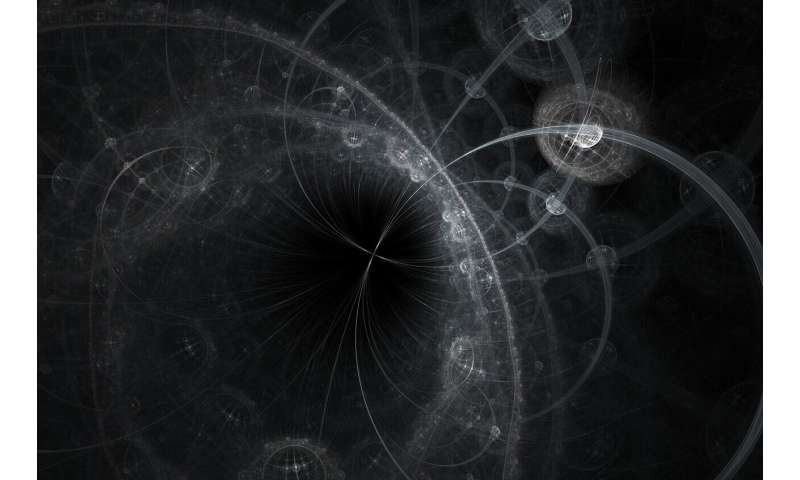
Credit: Pixabay / CC0 Public Domain
Atoms in a gas can look like partygoers at a nanoscopic rave, with particles whizzing around, mating, and flying again in seemingly random fashion. Yet physicists have come up with formulas that predict this behavior, even when atoms are extremely close to each other and can pull and pull at each other in complicated ways.
The environment within the nucleus of a single atom looks similar, with protons and neutrons dancing as well. But because the nucleus is such a compact space, scientists have struggled to define the behavior of these particles, known as nucleons, in the nucleus of an atom. Patterns describing the interactions of nucleons that are very far apart break down when particles mate and interact at close range.
Now a team led by MIT has simulated the behavior of protons and neutrons in different types of atomic nuclei, using some of the most powerful supercomputers in the world. The team explored a wide range of nuclear interaction models and found, surprisingly, that formulas describing how atoms behave in a gas can be generalized to predict how protons and neutrons interact at close range in the nucleus.
When the nucleons are less than 1 femtometer apart – 1 quadrillionth of a meter -, the researchers found another surprise: the particles couple in the same way, regardless of whether they inhabit a small nucleus like helium or a more crowded one like football.
“These short-haul couples don’t care about their environment, whether they’re in a big party or a group of five, it doesn’t matter – they’ll mate in the same universal way,” says Reynier Cruz-Torres, who co-led the work. as a graduate student in physics at MIT.
This short-range behavior is probably universal for all types of atomic nuclei, such as the much denser and more complicated nuclei in radioactive atoms.
“People didn’t expect this type of model to capture nuclei, which are some of the most complicated objects in physics,” says Or Hen, assistant professor of physics at MIT. “Despite a density difference of over 20 orders of magnitude between an atom and a nucleus, we can still find this universal behavior and apply it to many open problems in nuclear physics.”
The team published the results in the journal today Physics of nature. MIT co-authors include Axel Schmidt, a research affiliate of the Laboratory for Nuclear Science, along with collaborators from Hebrew University, Los Alamos and Argonne National Laboratories, and various other institutions.
Party couples
Hen tries to understand the disordered interactions between protons and neutrons at extremely short distances, where it has been notoriously difficult to define the traction between nucleons in the very small and dense environment of the nucleus. For years he has wondered whether a concept in atomic physics known as the contact formalism could also apply to nuclear physics and the inner workings of the nucleus.
In a very broad sense, the contact formalism is a general mathematical description that shows that the behavior of atoms in a cloud depends on their scale: those that are far from each other follow a certain physics, while atoms that are very close follow a completely separate set of physics. Each group of atoms carries out its own interactions unaware of the behavior of the other group. According to the contact formalism, for example, there will always be a number of ultraclose pairs, regardless of what other more distant atoms are doing in the cloud.
Hen wondered if the contact formalism could also describe interactions within the nucleus of an atom.
“I thought we couldn’t see this beautiful formalism, which was a revolution in atomic physics, yet we can’t make it work for nuclear physics,” says Hen. “It was just too much of a connection.”
“On a human scale”
The researchers first collaborated with Ronen Weiss and Nir Barnea at the Hebrew University, who led the development of a theoretical generalization of the atomic contact formalism, to describe a general system of interacting particles. They then sought to simulate particles in a small, dense nuclear environment to see if patterns of behavior would emerge among short-range nucleons, in a completely separate way from that of long-range nucleons as predicted by the generalized contact formalism.
The team simulated the interactions of particles within several light atomic nuclei, ranging from three nucleons in helium to 40 in calcium. For each type of atomic nucleus, they ran a random sampling algorithm to generate a movie of where each of the protons and neutrons in a given nucleus could be located over time.
“At a certain time shot, these particles can be distributed in a way, interacting with each other with a given pattern, where it couples with that, for example, and a third particle is kicked instead. Then, in another shot. temporal, they will be distributed differently, ”explains co-lead author Diego Lonardoni, a physicist at Los Alamos National Laboratory and Michigan State University. “So we repeat these calculations over and over to reach equilibrium.”
To see any kind of equilibrium, or pattern, emerge, the team had to simulate all the physics possible between each particle, generating thousands of snapshots for each type of nucleus. To perform this number of calculations would normally take millions of processing hours.
“My laptop would take longer than the age of the universe to complete the calculation,” says Hen. “If you spread the computation across 10,000 processors, you can get the result in a human-scale time.”
Then the team used supercomputers at Los Alamos and Argonne National Laboratory, some of the most powerful computers in the world, to distribute the work in parallel.
After running the simulations, they plotted a distribution of nucleons for each type of simulated nucleus. For example, for an oxygen nucleus, they found a certain percentage of nucleons within 1 stops of each other and another percentage slightly closer, and so on.
Surprisingly, they found that, for long-range nucleons, the distribution varied widely from one type of nucleus to another. But for short-range nucleons less than 1 femtometer apart, the distributions between atomic types looked exactly the same, no matter whether the nucleons inhabited an ultralight helium nucleus or a denser carbon nucleus. In other words, short-range nucleons behaved independently of their environment on a larger scale, similar to how atomic behavior is described through the contact formalism.
“Our discovery offers a new and simple way to define the short-range part of the nuclear distribution that, together with the existing theory, essentially allows for the complete distribution,” says Hen. “With this, we can test the nature of the neutrino and calculate the cooling rates of neutron stars, among other open questions.”
The light core was predicted to be stable despite having two strange quarks
Many-body factorization and position-moment equivalence of short-range nuclear correlations, Physics of nature (2020). DOI: 10.1038 / s41567-020-01053-7, www.nature.com/articles/s41567-020-01053-7
Provided by the Massachusetts Institute of Technology
Quote: Results on Short Range Nuclear Interactions Will Help Scientists Study Neutron Stars and Heavy Radioactive Nuclei (2020, November 9) recovered November 10, 2020 from https://phys.org/news/2020-11-short -range-nuclear-interactions -scientists-neutron.html
This document is subject to copyright. Apart from any conduct that is correct for private study or research purposes, no part may be reproduced without written permission. The content is provided for informational purposes only.
[ad_2]
Source link